Collaborative Virtual Reality for Surveying
Education
Dimitrios BOLKAS, Matthew O’BANION, Jeffrey CHIAMPI,
and Jordan LAUGHLIN, USA
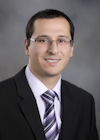 |
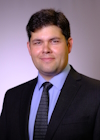 |
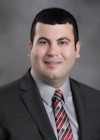 |
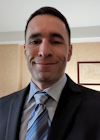 |
Dimitrios Bolkas |
Matthew O'Banion |
Jeffrey Chiampi |
Jordan Laughlin |
This article in .pdf-format
(15 pages)
This article is a peer-reviewed paper published and presented at the FIG Working
Week 2023 in Orlando, Florida. This paper presents virtual reality
implementations following two different pedagogical frameworks, the
first assessment results of collaborative learning and evaluate the role
of collaborative virtual reality to enhance student learning and support
surveying education.
Key words: virtual reality, surveying education, collaborative
learning, teamwork
SUMMARY
Immersive technologies have experienced rapid advancement in recent
years, and they have experienced widespread dissemination and
implementation in several disciplines including engineering. These
virtual reality implementations demonstrate a capability to support
education. However, implementations often lack connections with
theoretical pedagogical structures, leading to suboptimal results. This
paper presents virtual reality implementations following two different
pedagogical frameworks, namely experiential learning and situated
cognition / collaborative learning. In the former case students engage
in a virtual experience and learn by doing, while in the latter students
learn through communication and deriving solutions as a team. It is
widely accepted that teamwork and collaboration are increasingly
important skills in engineering. This paper demonstrates an example of
virtual reality labs in surveying engineering that follow a situated
cognition framework. The situated cognition labs are compared with
virtual reality labs that follow an experiential learning approach. The
paper presents the first assessment results of collaborative learning
and evaluate the role of collaborative virtual reality to enhance
student learning and support surveying education.
1. Introduction
Immersive technologies typically include Augmented Reality (AR),
Mixed Reality (MR), and Virtual Reality (VR). Immersive technologies
have experienced rapid advancement and development in the past decade,
with uses in the surveying profession and surveying education. In the
surveying profession it is worth highlighting Trimble SiteVision
(Trimble 2022) and the Leica vGIS (Leica-Geosystems 2022). These are AR
systems that allow accurate visualization of 3D models in the real
world, integrating 3D models with data collected in real time. Although,
this is an AR example, it shows how the industry is starting to utilize
immersive technologies. Virtual Reality, and immersive technologies in
general, have found more applications in education and training. Thanks
to their rapid advancement, we can find application examples in several
disciplines ranging from engineering, medicine and surgery, social
sciences, liberal arts, and more (examples of recent cases can be found
in: Chheang et al. 2021; Hur et al. 2021; Ma 2021; Singh et a. 2021). In
recent years, we also note several remarkable examples in surveying
education. For instance, O’Banion et al. (2020) used immersive
visualization to demonstrate the process of data acquisition from
airborne laser scanning. In Levin et al. (2020) students used a virtual
total station to collect topographic data to generate contours. Bolkas
et al. (2022) have developed software that allows students to collect
differential leveling data in an immersive and interactive VR. In Sakib
et al. (2020) an unmanned aerial vehicle (UAV) virtual training software
was developed to assist student preparedness with flying a UAV. We
notice that VR in surveying education is being used to prepare students
for hands-on labs, connect practical and theoretical concepts, develop
specific skills, and provide experiences that cannot be completed in the
real world due to cost, access, or liability limitations.
Virtual reality implementation should relate to an appropriate
theoretical framework and educators should have a solid rationale for
the use of VR and integration in existing curriculum (Johnston et al.
2018). Many of the existing VR implementations are often focused on
assessment of longstanding objectives not tailored to VR and
unfortunately the pedagogical structures are not clearly addressed
(Vincent et al. 2008; Solak and Erdem 2015; Johnston et al. 2018). The
lack of a robust link between VR application and pedagogical principles
and concepts leads to suboptimal implementations of VR in education
(Psotka 2013; Johnston et al. 2018). The pedagogical foundations that
are often found in VR, discussed in this paper, are derived by Kebritchi
and Hirumi (2008) and used in Johnston et al. (2018). These are briefly
described as follows:
- Direct instruction: students acquire skills through tutorials,
presentation of information, repetitive instructions, drill, and
practice;
- Experiential learning: students engage in real-life or virtual
experience; students observe, think, do and conceptualize and
experiment, learn by doing;
- Discovery learning: students build on existing knowledge to
learn new concepts through discovery, inquiry, applying
problem-solving and decision-making;
- Situated cognition: students are observers and actors, follow
others, engage in social interaction and communications to learn,
derive solutions as a team to problems; and,
- Constructivism: closely related to experiential and discovery
learning; knowledge is built by the learner, students gain knowledge
by making sense of experiences, students act, experiment, and
reflect within the experiences.
Of the above pedagogical foundations, experiential learning is often
cited in VR studies, and is the primary role in VR implementations. VR
opens the door to experience environments that are difficult to access,
dangerous, socially or culturally unacceptable, and/or very expensive
(Fowler 2015; Johnston et al. 2018). For this reason, Johnston et al.
(2018) also make note of secondary pedagogical foundations with their
analysis showing that discovery learning and constructivism appear as a
secondary role in most applications. This result is related to the
nature of role-play gaming where the gamer explores the environment and
discovers artifacts, clues, and information required to continue to the
next level or mission. Of particular note, there are few studies that
use situated cognition and collaborative learning as pedagogical
approach (Johnston et al. 2018). The same observation is found in
Potkonjak et al. (2016), who also analyzed many implementations of VR in
engineering education despite the implementations being desktop based
and not immersive. Many immersive VR implementations that utilize
situated cognition are from the military realm where soldiers are
trained as a team to respond in various threat situations (e.g., Bink et
al. 2015).
In situated cognition, knowledge is embedded in its context,
activity, and culture within which it is developed and used for learning
(Brown et al. 1989; Kebritchi and Hirumi 2008). The social interactions
and communications with others are fundamental to achieve learning
(Brown et al. 1989; Kebritchi and Hirumi 2008). Therefore, learners are
not isolated (as they are in many VR implementations); rather, they
learn while interacting with other students within shared activities
that are designed to facilitate communication, discussion,
problem-solving, transfer of knowledge, and skills (Aydede and Robbins
2009). Situated cognition is based on social development theory where
social interactions are the main method for developing cognition
(Vygotsky 1978). The activity used as means for learning is also an
important component of the theory of situated cognition, as activities
must be authentic and framed by the domain’s (or profession’s) culture
and ordinary practices (Brown et al. 1989). Concepts such as teamwork,
engaging in technical and diverse discussion, learning from peers and/or
instructors, and collaborative learning are integral to engineering and
essential aspects of Accreditation Board for Engineering and Technology
(ABET) accreditation for many surveying / geomatics programs.
Collaborative learning is an integral aspect of situated cognition, and
it can be achieved by designing activities and labs that have situated
cognition as their primary pedagogical focus.
Situated cognition provides the means for context-based collaborative
learning, transferring of knowledge and skills between learners, and
simulates real-world learning settings. Immersive technologies become
the “next big thing” and an important tool for many engineering
disciplines (Piroozfar et al. 2018); therefore, integrating such
technologies in the surveying curriculum is vital for preparing future
surveyors. This paper demonstrates an example of situated cognition
implemented through VR surveying engineering labs. The VR labs are
designed under the situated cognition framework, and compared with VR
labs that follow an experiential learning approach. The main research
question is: can situated cognition support and assist learning of
surveying engineering principles in activities that are designed in
immersive and interactive VR? The paper presents the first assessment
results of situated cognition / collaborative learning and evaluates the
role of collaborative VR to enhance student learning and support
surveying education.
2. VIRTUAL REALITY LABS
The VR labs that were used in this paper were on differential
leveling and using the Surveying Reality (SurReal) software that was
presented in Bolkas et al. (2021). The software simulates differential
leveling in immersive and interactive VR. It was expanded to include
GNSS labs, and, in the future, is expected to support exercises that
utilize a total station instrument. The software uses the Oculus Rift
for controls and for the head mounted display (HMD), and it can also use
similar Oculus devices that can connect to a desktop computer such as
the Oculus Quest. The software and instrument handles were developed in
Unity, while several of the 3D models and buildings were developed in
Autodesk 3DS Max and Blender (see Bolkas et al. 2020; Bolkas et al.
2021). Students can grab and move the differential level instrument,
level it by adjusting the legs and tribrach screws, and make
measurements and record them in a virtual fieldbook (Bolkas et al. 2021;
Bolkas et al. 2022). The lab is based on a three-benchmark leveling loop
(Figure 1), where for each setup we increase the level of difficulty to
challenge the student (Bolkas et al. 2022).
Figure 2 shows a side of the benchmark locations. In the first
segment (BM1 to BM2), there is no real challenge, as we want to give
time to the student to get familiar with the software and the VR
controls. In the second segment (BM2 to BM3), there is a road sign next
to BM2, which can block the line of sight; therefore, the student needs
to select a suitable instrument location. In the third segment (BM3 to
BM1), we have added a car as an obstacle, there is high terrain
variability, and tree branches can block the view. If the student does
not select an appropriate location to setup the instrument, then the
student will either aim to low at BM1 or the view to BM3 will be blocked
by trees.
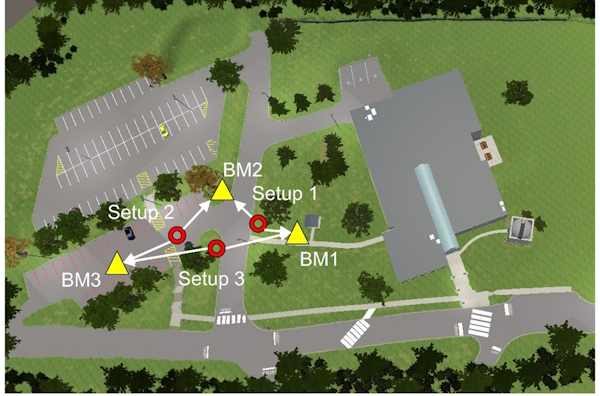
Figure 1. Virtual environment and virtual lab. The figure shows the
benchmark (BM) locations and example instrument setups.
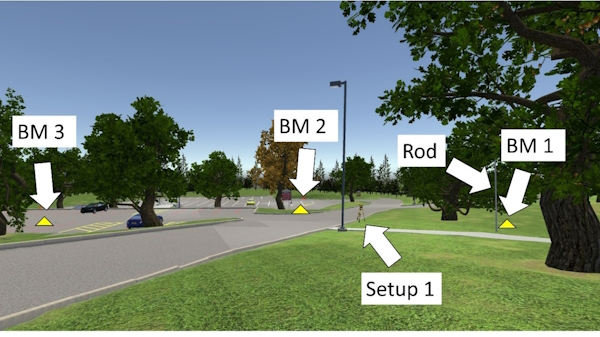
Figure 2. Ground level perspective view of the benchmark locations in
the three-benchmark leveling loop.
2.1 Experiential learning approach
In the experiential learning approach, the students complete the virtual
lab on their own, meaning that they are the only surveyor in their
specific virtual lab. The focus of the virtual experience is to prepare
students for the physical lab by allowing the students to learn by
doing. Prior to the virtual lab, the students receive theoretical
instruction on differential leveling through traditional means i.e.,
class demonstrations and presentations. In the virtual experience, the
instructor demonstrates the main controls and the process of completing
a differential leveling loop in VR. Students then conduct the lab in VR
on their own, handling both the differential level instrument and the
leveling rod. This approach has the advantage of exposing the student to
both the instrument and rod roles, giving them a complete understanding
of the differential leveling process. Therefore, in the experiential
learning approach students engage in the virtual experience, and in
general learn by doing. In addition to the experiential learning, the
virtual lab also has elements of discovery learning, as students build
knowledge by problem solving, decision making, inquiry, and trial and
error. For instance, consider the student making decisions about the
optimal position of the differential level considering terrain and line
of sight constraints. At the end of the experience, students receive a
report of their observations along with the true observations generated
by the software. This introduces elements of constructivism, as students
can identify their mistake and make sense of their experience and
decisions.
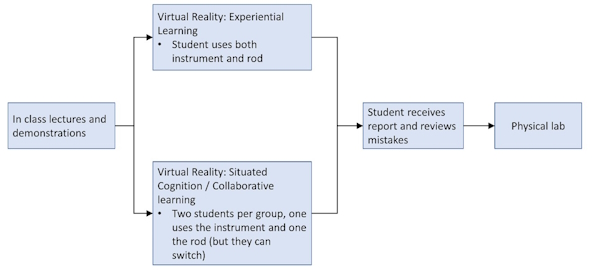
Figure 4. Flowchart of the experiential learning and the situated
cognition implementation approaches
2.2 Situated Cognition / Collaborative Learning
For the situated cognition approach, the focus of the lab is to use
collaboration, communication, and interaction to learn and derive
solutions as a team. The group of students that completed the
collaborative virtual lab followed the same surveying process as the
individual student group; however, two students were able to co-exist in
the same virtual environment and work together. For each team of two,
one student assumed a leadership role and operated the leveling
telescope instrument, while the other took a follower role and handled
the leveling rod (Figure 5). Instructions about the leader-follower
roles were emphasized before conducting the virtual lab. Participants
were able switch roles at any point throughout the virtual exercise and
were encouraged to do so following each instrument setup. Following
their collaborative completion of the differential leveling loop, each
team was able to review the exercise performance report where they can
identify any mistakes made and discuss possible mitigation strategies.
As mentioned above, the focus of the virtual exercise is for students to
learn through collaboration, communication, and interaction; however, it
is important to highlight that the exercise still maintains elements of
experiential learning, discovery learning, and constructivism. A
disadvantage of this method is that the students experience only one
role if they do not switch; however, this can take place in the
real-world as well. To counter this in the virtual world, a forced role
change could be implemented.
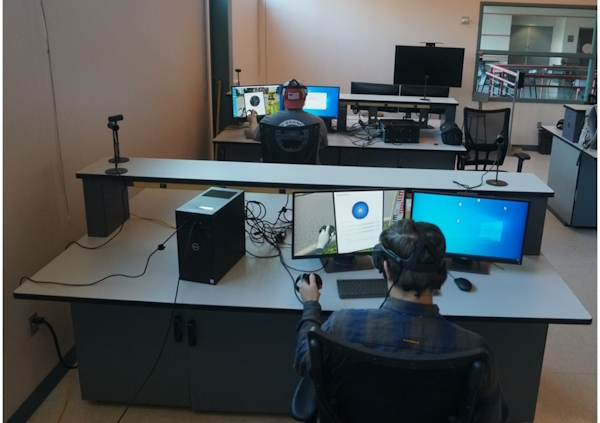
Figure 5. Students collaboratively complete a virtual lab in SurReal.
The student in the background is handling the differential level
instrument, while the student in the foreground is handling the leveling
rod.
2.3 Student Sample and Assessment
The two experiential and situated cognition labs were implemented at
Penn State Wilkes-Barre in an introductory surveying course in Fall of
2021. In addition, the experiential learning labs were implemented at
the United States Military Academy (USMA) at West Point, New York. The
situated cognition labs were not operational at the time of the USMA
trials due to a technical issue with the multiplayer SDK of the
software. Therefore, the focus is placed on the Penn State Wilkes-Barre
sample. There were 14 students in SUR 111, the introductory surveying
course at Penn State Wilkes-Barre, in Fall of 2021. Of those students, 8
were randomly selected to complete the virtual leveling lab in the
situated cognition framework; thus, forming 4 groups of 2 students. The
remaining 6 students completed the virtual leveling lab in the
experiential learning framework.
To assess the two learning approaches, students completed pre- and
post-tests. The pre-test had questions related to trigonometry such as
calculating angles and distances in a right triangle using the sine,
cosine, and tangent formulas, using the law of cosines, and the
Pythagorean Theorem. The post-test, was a midterm exam, and we focus on
a question that asked students to prepare a set of leveling notes,
calculate the page check, and calculate the adjusted elevation for one
BM. The leveling line had three leveling setups with three backsight and
three foresight measurements. In addition to the post-test, we use the
student performance during two physical leveling labs at Penn State
Wilkes-Barre that followed the virtual lab.
Assessment using pre- and post-tests may not be the most suitable
measure to show learning differences and differences related to teamwork
and collaboration. Therefore, we augment our assessment with
student-to-student evaluations about their collaboration. This
evaluation is concentrated around a three-question survey where students
were asked to rate their partners with scores ranging from 1 to 5:
- Demonstrates good and
encourages communication among teammates;
- Demonstrates
participation in decision making;
- Demonstrates active
team member participation in assigned-role duties.
Furthermore, after the students completed the situated cognition virtual
lab they answered the following questions, again providing scores
ranging from 1 to 5:
- I liked the ability to
communicate in the VR lab;
- Rate the communication
quality;
- Rate the collaboration
with your partner;
- Working in a group
helped me learn;
- Working in a group in
VR was enjoyable.
3. RESULTS
3.1 Student Background
Table 1 provides information about the background of students with
respect to surveying and VR. Almost all students in both samples have no
prior experience with surveying and the differential leveling process.
In terms of their experience with VR, 4 students in the situated
cognition and 2 students in the experiential learning samples had used
VR before. However, their experience was very minimal, and they
indicated that they had used VR only a few times as part of a course or
for gaming purposes. Both samples can be considered as non-experienced
with both surveying and VR. This is important and worth highlighting
because it makes the process of learning using immersive technologies
challenging. Students have to learn VR and then be able to conduct the
virtual lab; therefore, sometimes their ability to learn how to use VR
can affect their learning and progress in the virtual lab. Compared to
previous years, we find that an increased number of students have had
some exposure to immersive visualization technologies, which is
encouraging for future virtual lab implementations, as students may come
more prepared to use this technology and be able to focus on the virtual
task / lab.
Table 1. Sample background and experience with surveying and VR

3.2 Virtual Leveling Lab Results
First, we evaluate the virtual leveling lab results in terms of their
misclosure and ability to balance the backsight and foresight distances
(Table 2). Of the 4 groups in the collaborative student sample, two
achieved a misclosure of 5 mm or less. While two groups had higher
misclosures at the level of a few decimeters. For the one group, this
was due to a blunder measurement. For the other group, it was because
one student forgot to level the instrument in one setup. In
contrast of the six students conducting the lab on their own, two
students achieved a misclosure of less than 3 mm, two students achieved
a misclosure of 2-4 cm, and two students did not finish the lap. The
moderate accuracy (2-4 cm) of the two students was due to poor leveling
of the instrument. The two students that did not finish the lab was
because they exceeded the time allowed (i.e., more than 60 minutes). We
notice that the situated cognition group needed less time to complete
the labs than the experiential learning group. In the situated cognition
group the tasks are shared; however, in the experiential learning lab
students need to level the instrument and then the rod, thus needing
more time to complete the lab. Of note is that several students of the
experiential learning group did not follow the suggested format for
recording measurements, which is not the case for the situated cognition
student sample. It was observed that the situated cognition students
communicated on how to set up the fieldbook, record the measurements and
therefore, followed the suggested format. Some students in the
experiential learning sample who did not know how to properly record
their measurements hesitated to ask the instructor for assistance and
ended up with incorrect fieldbook formats. After the virtual lab, the
instructor showed the report to the students, and discussed the mistakes
and how to improve their surveying in the future. In the physical lab
(introduction to leveling), where students complete a three-benchmark
loop similar to the virtual lab, all groups achieved the required
misclosure in their first attempt, and all groups followed the correct
fieldbook format. This demonstrates the ability of both VR approaches to
prepare students for the physical lab.
Table 2. Virtual lab statistics. There are 6 students in the
experiential learning lab and 4 groups (8 students) in the situated
cognition lab.
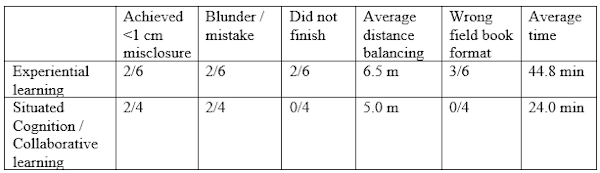
Table 3. Student feedback related to general pedagogy and surveying
pedagogy questions. Average scores are shown (Scores range 1 to 5).
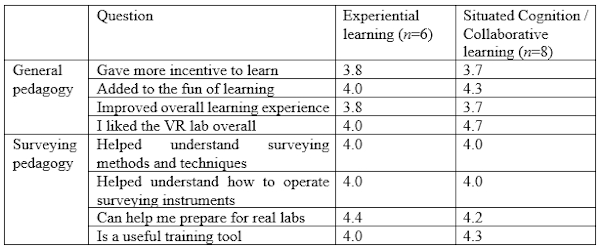
Table 4. Student feedback of the situated cognition virtual lab related
to their ability to work in a group and communicate in VR. Average
scores are shown (Scores range 1 to 5).
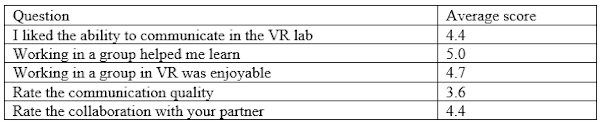
Table 3 shows the student feedback related to the pedagogical
contributions of VR. Both groups provided similar feedback and they
indicate that VR helped students learn, improve their learning
experience, understand surveying techniques, and helped them prepare for
the physical labs. Furthermore, the student group who conducted the
situated cognition labs expressed their strongly positive feedback for
the ability to work in groups in VR, and that working in a group it
helped them learn (Table 4).
3.3 Pre-test and Post-test Assessment and Peer-to-Peer Evaluation
The average pre-test grade for the experiential learning and situated
cognition groups were 43.1% and 46.0%, respectively for the two groups.
Statistically they are not significantly different, as the t-value is
-0.197; the p-value is 0.848; therefore, the result is not significant
at p <0.05. This indicates that there is no inherent bias between the
test and control groups. The scores of the post-test question were 88.9%
and 88.1%, for the experiential learning and situated cognition groups
respectively. The post-test question was given as part of the class
midterm exam. The overall grades of the midterm exam, which contained
leveling and other non-leveling questions, were very similar as well
i.e., 90.4% and 88.9%, respectively. The scores of the introduction to
leveling lab, where students complete a three-benchmark loop similar to
the virtual lab, were 92.5% and 95.0%, respectively. While for the
second differential leveling lab, which is a leveling circuit starting
from one benchmark and ending at a different benchmark, the scores were
95.0% and 95.6%, respectively. A notable difference was found with
respect to the peer-to-peer evaluations. Table 5 shows the average
scores for three questions asked in each group. We notice higher scores
for the situated cognition group. Q1 and Q3 yield statistically
significant differences; therefore, indicating a positive effect of the
situated cognition labs in student collaboration.
Table 5. Peer-to-peer student evaluations related to their teamwork and
collaboration in the physical lab.
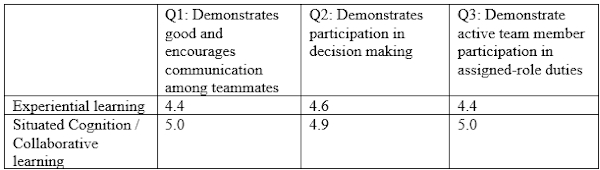
3.4 Comparison with Previous Years
In Table 6, we show a comparison of average grades for the midterm exam,
which contains several leveling questions, and two outdoor differential
leveling labs. Years 2019 and 2021 are years where students conducted VR
labs. In 2019 all students conducted the experiential learning lab, and
in 2021 6 students conducted the experiential learning lab and 8
students the situated cognition lab. Some years are missing student
grades due to instructor turnover. In general, we note the positive
effect in student grades when VR is used. The three-benchmark loop lab,
which is conducted a week after the virtual lab, seems to have the most
benefit of the virtual labs. Students are better prepared for the
physical lab; having conducted a very similar virtual lab first.
Table 6. Average grades in related exams and assignments. 2019 and 2021
are the years where VR technology was used. In 2019 an experiential
learning approach was used. In 2021 students were separated into an
experiential learning and a situated cognition group.
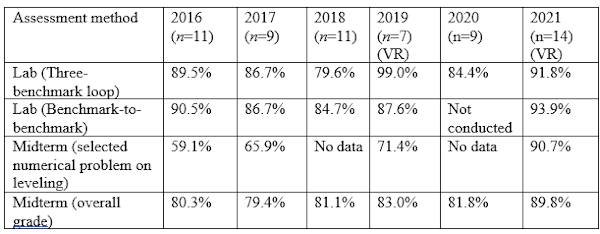
The results of this paper demonstrate that both experiential learning
and situated cognition / collaborative learning approaches are suitable
for preparing students for physical labs. In both approaches the lab and
exam scores were higher compared to the years without VR, indicating
that both methods can be used to enhance learning. The main difference
between the two virtual learning approaches is in the student
satisfaction of their collaboration during the physical labs. These
first results show that a collaborative VR approach is able to enhance
their teamworking skills and help students prepare for a successful
collaboration in the physical labs (Table 6). However, additional
implementations in larger student populations are needed to further
understand the differences of experiential learning and situated
cognition, and the role of the latter approach in surveying engineering
education.
4. CONCLUSIONS
Surveying engineering is a profession that continuously experiences and
embraces new technological advancements. Virtual reality technology has
found its way into the surveying profession and surveying education.
With respect to the latter, we see in an increased number of case
studies testing and integrating immersive technologies in surveying
engineering education. It is important that the integration of immersive
technologies takes place under a theoretical framework to identify and
attain specific pedagogical goals. This study presented the pedagogical
foundations that we often encounter in immersive technologies. We have
presented and implemented VR labs that followed two different
approaches, namely experiential learning and situated cognition /
collaborative learning. In the former approach students engage in a
virtual experience and learn by doing, while in the latter the focus is
placed on communication and collaboration. The assessment of the two
approaches showed that both immersive implementations are capable of
supporting surveying engineering education and assisting in preparing
students for physical labs. We find no significant difference between
the two VR implementations; however, comparison with years without the
support of VR, shows a significant difference in favor of the VR
technology. We observed a significant difference in the peer-to-peer
evaluations in terms of the teamwork and collaboration in the physical
labs, indicating that the situated cognition approach is able to also
enhance teamwork skills. Despite the many benefits of conducting
collaborative VR labs, we do find an important limitation. The
multiplayer support in VR makes the implementations more complex, and
there is higher potential for software issues, which we experienced in
the USMA implementation. There are also challenges related to hardware,
software, and driver updates; thus, maintaining a VR system for teaching
is still demanding. In future years, we plan to conduct additional tests
and using larger student samples to further explore and understand how
the two different learning approaches can support surveying engineering
education.
REFERENCES
- Aydede, M., & Robbins, P. (Eds.). (2009). The Cambridge handbook of
situated cognition. New York, NY: Cambridge University Press
- Bink, M. L., Injurgio, V. J., James, D. R., Miller, I. I., & John, T.
(2015). Training Capability Data for Dismounted Soldier Training System
(No. ARI-RN-1986). Army Research Inst For The Behavioral And Social
Sciences Fort Belvoir Va.
- Bolkas, D., Chiampi, J., Chapman, J., & Pavill, V. F. (2020). Creating a
virtual reality environment with a fusion of sUAS and TLS point-clouds.
International journal of image and data fusion, 11(2), 136-161.
- Bolkas, D., Chiampi, J., Fioti, J., & Gaffney, D. (2021). Surveying
reality (SurReal): Software to simulate surveying in virtual reality.
ISPRS International Journal of Geo-Information, 10(5), 296.
- Bolkas, D., Chiampi, J. D., Fioti, J., & Gaffney, D. (2022). First
assessment results of surveying engineering labs in immersive and
interactive virtual reality. Journal of Surveying Engineering, 148(1),
04021028.
- Brown, J. S., Collins, A., & Duguid, P. (1989). Situated cognition and
the culture of learning. Educational researcher, 18(1), 32-42.
- Chheang, V., Saalfeld, P., Joeres, F., Boedecker, C., Huber, T., Huettl,
F., Lang, H., Preim, B. & Hansen, C. (2021). A collaborative
virtual reality environment for liver surgery planning. Computers &
Graphics, 99, 234-246.
- Fowler, C. (2015). Virtual reality and learning: Where is the
pedagogy?. British journal of educational technology, 46(2), 412-422.
- Hur, J. W., Shin, H., Jung, D., Lee, H. J., Lee, S., Kim, G. J., Cho,
C.Y., Choi, S., Lee, S.M. & Cho, C. H. (2021). Virtual reality–based
psychotherapy in social anxiety disorder: fMRI study using a
self-referential task. JMIR mental health, 8(4), e25731.
- Johnston, E., Olivas, G., Steele, P., Smith, C., & Bailey, L. (2018).
Exploring pedagogical foundations of existing virtual reality
educational applications: A content analysis study. Journal of
Educational Technology Systems, 46(4), 414-439.
- Kebritchi, M. & Hirumi, A. (2008). Examining the pedagogical foundations
of modern educational computer games. Computers & Education, 51(4),
1729-1743.
- Leica-Geosystems (2022). vGIS.
https://leica-geosystems.com/en-us/products/gis-collectors/gis-partners/vgis
[Accessed 12/31/2022]
- Levin, E., Shults, R., Habibi, R., An, Z., & Roland, W. (2020).
Geospatial virtual reality for cyberlearning in the field of topographic
surveying: Moving towards a cost-effective mobile solution. ISPRS
International Journal of Geo-Information, 9(7), 433.
- Ma, L. (2021). An immersive context teaching method for college English
based on artificial intelligence and machine learning in virtual reality
technology. Mobile Information Systems, 2021.
- O'Banion, M. S., Majkowicz, D. C., Boyce, M. W., Wright, W. C.,
Oxendine, C. E., & Lewis, N. S. (2020). Evaluating immersive
visualization technology for use in geospatial science education.
Surveying and Land Information Science, 79(1), 15-22.
- Piroozfar, A., Farr, E. R., Boseley, S., Essa, A., & Jin, R. (2018). The
application of Augmented Reality (AR) in the Architecture Engineering
and Construction (AEC) industry. In Proceedings of the 10th
International Conference on Construction in the 21st Century (CITC-10),
July 2-4, Colombo, Sri Lanka.
- Potkonjak, V., Gardner, M., Callaghan, V., Mattila, P., Guetl, C.,
Petrović, V. M., & Jovanović, K. (2016). Virtual laboratories for
education in science, technology, and engineering: A review. Computers &
Education, 95, 309-327.
- Psotka, J. (2013). Educational games and virtual reality as disruptive
technologies. Journal of Educational Technology & Society, 16(2), 69–80.
- Sakib, M. N., Chaspari, T., Ahn, C., & Behzadan, A. (2020, July). An
experimental study of wearable technology and immersive virtual reality
for drone operator training. In Proceedings of the EG-ICE 2020 Workshop
on Intelligent Computing in Engineering, Berlin, Germany (pp. 1-4).
- Singh, G., Mantri, A., Sharma, O., & Kaur, R. (2021). Virtual reality
learning environment for enhancing electronics engineering laboratory
experience. Computer Applications in Engineering Education, 29(1),
229-243.
- Solak, E., & Erdem, G. (2015). A Content Analysis of Virtual Reality
Studies in Foreign Language Education. Participatory Educational
Research, spi15, 2, 21-26.
- Trimble (2022). Trimble Sitevision.
https://sitevision.trimble.com/ [Accessed 10/13/2022]
- Vincent, D. S., Sherstyuk, A., Burgess, L., & Connolly, K. K. (2008).
Teaching mass casualty triage skills using immersive three‐dimensional
virtual reality. Academic Emergency Medicine, 15(11), 1160-1165.
- Vygotsky, L. S. (1978). Mind in society. Cambridge, MA: Harvard
University Press.
BIOGRAPHICAL NOTES
CONTACTS
Dr. Dimitrios Bolkas
Pennsylvania State University, Wilkes-Barre Campus
44 University Drive
Dallas, PA, 18612
United States of America
Tel. +1 570 675 9127
Email: dxb80 [at] psu.edu
Dr. Matthew O’Banion
United States Military Academy
745 Brewerton Rd.
West Point, NY, 10996
United States of America
Email: matthew.obanion [at] westpoint.edu
Jeffrey Chiampi
Pennsylvania State University, Wilkes-Barre Campus
44 University Drive
Dallas, PA, 18612
United States of America
Tel. +1 570 675 9237
Email: jdc308 [at] psu.edu
Jordan Laughlin
United States Military Academy
745 Brewerton Rd.
West Point, NY, 10996
United States of America
Email:
jordan.laughlin [at] westpoint.edu